攻殻機動隊 M.M.A. - Messed Mesh Ambitions_
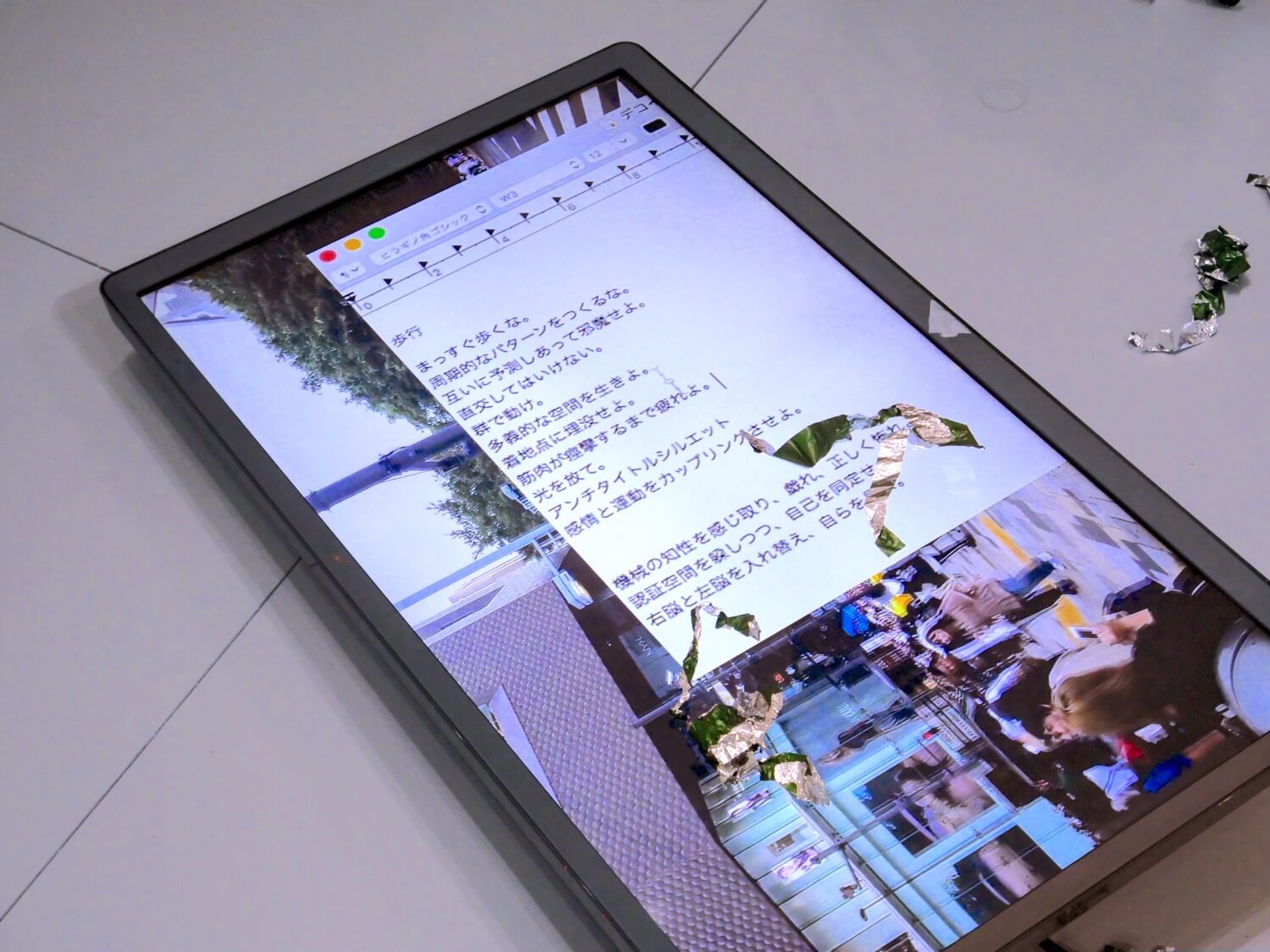
Goro Murayama, Decoy-walking, 2019
Taming “out-and-out muddle”—Swarm as ambiguous Body, or body as ambiguous swarm
[Credits]Text_Hisashi Murakami
Images_Hisashi Murakami, Goro Muyayama
The cars are packed so densely it’s as though leaving space is an alien concept. Drivers make sudden lane changes, merging across six lanes of traffic on a four-lane street. To Japanese sensibilities, all of the drivers seem entirely composed as they narrowly avoid accidents at the last moment amidst this chaos (although of course, accidents must be happening, statistically speaking). Though it looks completely uncoordinated at first glance, apparently there is a cohesive organization to it. Faced with such a mysterious scene, one can’t help but ask a question.
How is this sort of situation possible?
On that point, Hisashi Murakami—a researcher who uses mathematical models of animal swarming behavior for analytical purposes—can offer a few persuasive answers. What does it take to make a swarm with individual dynamics? Swarms aren’t just formed for the purpose of surviving outside disturbances. Once one understands that they also require internal disturbances to exist, it becomes easier to see the hidden order underlying the chaos of those six lanes of traffic. The outlaw organization Public Security Section 9 is no exception to that rule.
As Sakae Otsugi would put it, there is “beauty in disarray.” Or as Murakami might say, “there is order in chaos.”
[Contents]
- Out-and-out muddle
- My Own Body, and Yet Not
- Collective Animal behavior
- Swarms of soldier crabs, Schools of Sweetfish
- Asynchrony and Mutual Anticipation
- Swarm Formation as a Structure with Consensual Breakdown
- Human Swarms: Introduction to Mutual Anticipation
- Human Swarms: Introduction to Asynchrony
- Swaying Swarms
- “You’d be amazed how much it takes for me to be myself”
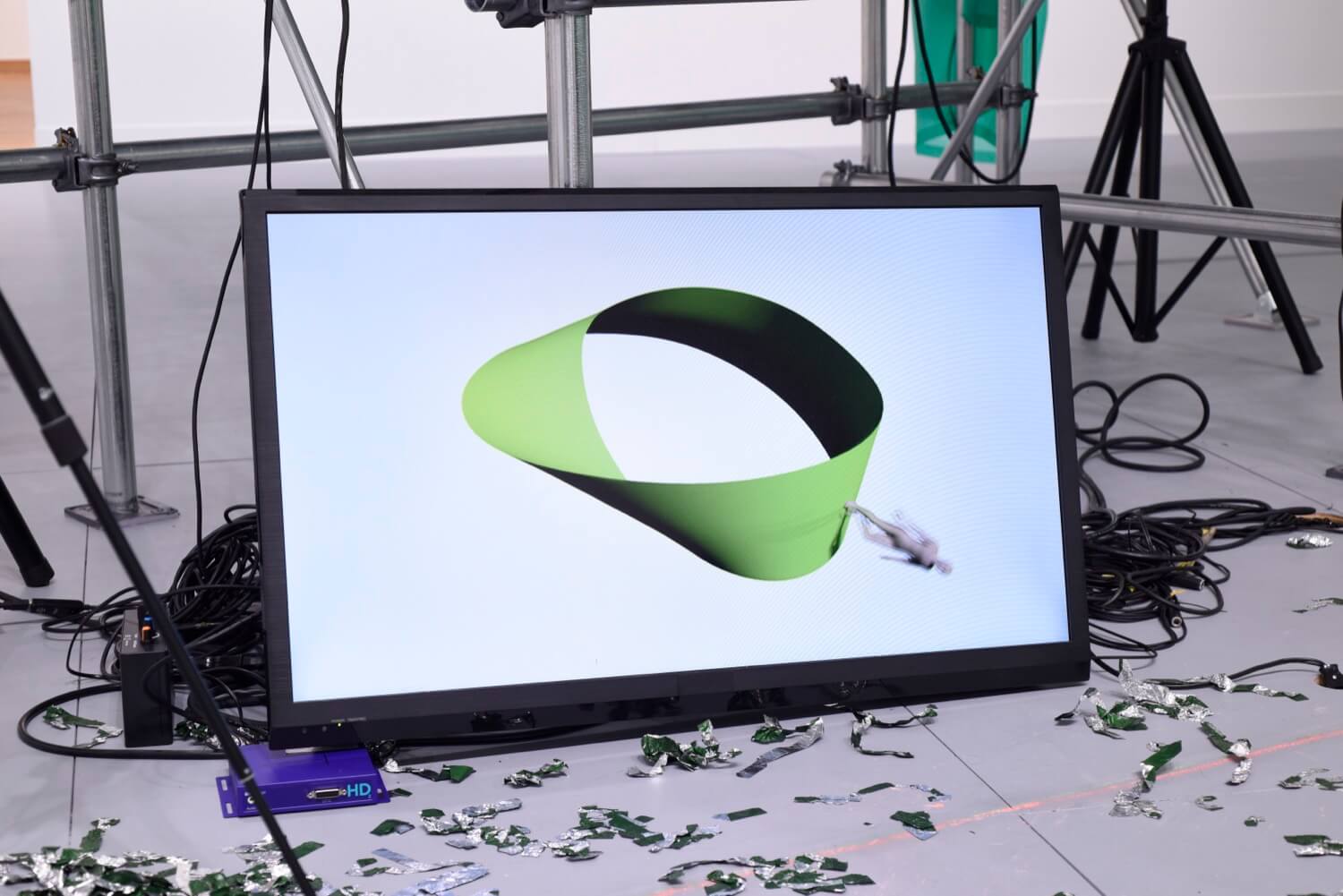
Goro Murayama, Exchange the left and right brains and look at yourself., 2019/Photo by Tetsuo Ito
Out-and-out muddle
Father: So he said they should use flamingos as mallets because the flamingos would bend their necks so the player wouldn’t know even whether his mallet would hit the ball….Daughter: Anyhow the ball might walk away of its own accord because it was a hedgehog. … Did everything have to be alive so as to make a complete muddle?Father: No… I suppose you’re right. … once you bring live things into it, it becomes impossible. I wouldn’t have expected that.-Gregory Bateson, “Metalogue: Why Do Things Have Outlines?”
As “a real concrete out-and-out muddle”, Bateson puts forth the idea of a game of croquet (a type of ball game) with flamingos in place of mallets and hedgehogs in place of balls. Flamingos and hedgehogs move on their own accord regardless of the croquet player’s intent. The time difference between the decisions of each player and the equipment creates chaos. The creatures (the flamingos and hedgehogs) each live on their own distinct time, and adding them as equipment to the game transforms croquet into something entirely unpredictable for the players, who otherwise could compete based on predictable techniques. This changes the nature of the game.
Bateson likens the difference in species, such as humans, flamingos, and hedgehogs, to the time differences experienced between them. But there is no need to analyze inter-species croquet, as the same type of time discrepancies can be found in the routine behavior of single species groups. There is no single clock shared across entire swarms of birds or fish; each individual operates on its own time frame. Despite the infinite number of misalignments this generates, these swarms behave with such unity as to seem like single creatures. This is what leads to the question: is out-and-out muddle always lurking under the surface of organized living groups.
My Own Body, and Yet Not
“The system” in this case refers to the Major’s body, which is entirely cybernetic aside from the brain. In other words, the concept extends even to systems of an individual body. The Major’s cybernetic body, composed of standard parts, would also presumably be more controllable than a flesh-and-blood human body. However, the system cannot attain perfection due to the integration of the non-standard brain, which contains unexpected deviations. These deviations cannot be reduced to the discomfort of a mind-body dualistic identity, which arises when the “me” feels that “this is not my body.” To the contrary, the displacement the Major senses may be the fact that systems require heterogeneity to function, something she has seen proven on the smaller scale of the individual body.
The deviations in the system of her body may point the Major toward an existential issue, as they prevent her body’s complete integration as a system and erode the basis for viewing herself as a single, closed entity. This leads her to a view more common of a full-body cyborg: “Maybe there never was a real me in the first place.” But even as she speaks of this existential anxiety, when asked why someone would dive into the sea (vast and unknowable as it is) in comparison with a pool (which is fully controllable), she responds, “Fear, uncertainty, loneliness, doubt, and maybe even hope. They all change you when they come to the surface.” Her words speak to the fear of not being able to secure one’s identity in a closed system, and a hopeful openness to change. They are two sides of the same coin. And in fact, once the grounds for the individual’s existence are established, change seems unlikely. Conversely, in order to change, existential anxiety must be present. There can be no established grounds for the individual’s existence. This ambiguity of the individual as a system seems closely related with the existential anxieties and identity displacement arising in cyborg bodies.
However, being a full-body cyborg comprised of standardized parts—except for her brain—in the highly developed future society depicted in Ghost in the Shell, would not be the only thing that would make the individual system discomfort. This is because even flesh-and-blood humans feel a lurking alienness beneath the surface of the system of the self. We typically think our conviction that “this is my own body” is unshakable. (This is called our sense of bodily ownership.) However, like a rubber illusion or a full out-of-body experience, this sense of bodily integration warps quite easily where it had once seemed self-evident with just a slight change of parameters*1. Moreover, even for non-cybernetic beings, one can experience the ambiguous sensation of our bodies being both our own and not our own. To put it simply, subjects observing their own bodies from the side with a head-mounted display will feel “that [they] are the one moving [their] arms (a sense of agency) yet also a sense that these are not their arms (disownership)” just by hiding their elbows behind their bodies from the displayed POV*2. A cyborg consisting of a fusion of mechanical and organic material might experience even more significant discomfort and disjunctions, but in reality, even a flesh-and-blood human can feel this sensation under certain conditions. Rather, the adaptability of the bodily system to accept foreign matter as is may itself be a condition required for prosthetization—or artificial modification—of the body after birth.
Neither individuals nor collectives can fully eliminate displacement and discomfort from the organizational processes of their systems. Moving on, we’ll look at how displacements and discomforts inherent in biological systems in the natural world are dealt with.
Goro Murayama, Decoy-walking, 2019
Collective Animal behavior
The question of what makes individuals and collectives behave as they do has been a longstanding question, as people have examined relationships between the part and the whole. For example, late 19th and early 20th century ornithologist, Edmund Selous, who was a proponent of Dawin’s theory of evolution, conducted a lifelong study of grey starlings, and argued that their unified behavior in flocks of tens of thousands showed that there was some form of direct connection between individual birds, and that the behavior would be impossible without the direct communication of thoughts*3. Modern researchers believe that collective animal behaviors are achieved through a full activation of a variety of senses (not telepathy), but it took until the late 20th century for science to advance on this front.
It was in 1987 that artificial life researcher Craig Reynolds constructed a mathematical model using swarms called “Boids” (or “bird-oids”) to reproduce this behavior in CG animation*4. Individuals in this model interact with other individuals in a set radius around them, following three rules of “alignment” via heading averaging, “cohesion” to avoid leaving the swarm, and “separation” to avoid colliding other individuals. Just setting these three simple rules let the Boids reproduce swarm-like cohesive movements on a computer, and they have been used as the algorithm for crowd movement in films like Batman Returns.
Following Reynolds’ work, while various mathematical models similar to Boids have been proposed using biological perspectives distinct from CG animations, almost all use the same rule of average headings. For example, research involving heading averages as a minimal principle for swarm formation while applying an external disturbance that adds some level of diffusion to individual headings is popular even in the realm of statistical physics modeling of physical particle swarms*5. Even when models are made using only heading averaging as a principle, individual particles will form massive swarms rapidly as accidental collisions between individuals produce aligned trajectories. However, this alone results in a collective where all members continually flow in one direction, thus lacking dynamism and flexibility. As such, uniform disturbances from outside the swarm are added to increase the individual freedom to act. Of course, if the external disturbance is too severe, then the individuals scatter, and cannot form a swarm. The resulting models form representations of phase transitions with the external disturbance as a parameter value for the critical point of transition from a solid phase (complete order) to a liquid phase (complete chaos). Actual biological swarms are believed to form in the critical point between two polars. One could contend that in mathematical models like Boids that define cohesion as a swarm (alignment) as sociality, there is a tendency to posit the freedom of movement that draws individuals away from the collective as a vector, with an opposing vector of the sociality that organizes the swarm.
The late 2000s also saw dramatic improvements in a variety of computing and tracking systems and technologies, letting researchers analyze animal swarms in the natural world in detail. In particular, stereo camera photography of flocks of thousands of grey starling birds revealed several phenomena that the conventional Boids mathematical models could not explain when researchers used image analysis to reconstruct bird coordinates in three dimensions. For example, examination of the spacial distribution of the fluctuation vector of individual birds (the difference between the flock’s average velocity and an individual flight velocity) showed correlations of fluctuation vectors among individuals within the flock, revealing multiple areas where individuals shared a type of information. Surprisingly, this range of spatial correlation grows in a linear fashion in direct proportion to the size of the entire swarm. This phenomenon is called scale-free correlation*6. This was astonishing because a short range of interaction, assumed by most models to simulate self-organizing phenomena in animal groups, is usually considered to result in a short range of correlation. However, in actual swarms the correlative area within the swarm grows with the swarm itself, just like a creature with a single body. When considering the form that living swarms would take in the field under the opposing relationship seen in Boids-type mathematical models between sociality—meaning a uniform collective structure—and the free individual movement that results from a uniform external disturbance, we would inevitably move toward hypothesizing uniform disturbances to individual flight speeds applied from outside the swarm, similar to disturbances to the principle of heading alignment. Accordingly, the fluctuation vector would also be caused by an external disturbance, and subject to the randomness that guides chaotic swarm behavior. Such a vector cannot explain the presence of correlative areas generated by individual disturbance factors within the flock. Conventional models (in which sociality from alignment and free individual movement from external disturbances are in opposition to one another) struggle to explain the real-world observations that show these correlative areas. This is because disturbances or noises in actual swarms are inherently generated.
A group of soldier crabs (Mictyris guinote) walking on Funaura Bay tidal flat in the northern part of Iriomote Island/Photo by Hisashi Murakami
Swarms of soldier crabs, Schools of Sweetfish
These observations are not exclusive to soldier crab swarms. The same is true for flocks of birds and schools of fish, in that they all appear precisely aligned and orderly at a glance. Our team examined juvenile sweetfish schools*9for analysis*10. First, 10 to 60 sweetfish juvenile were released into an experimental tank. Their collective behavior was then recorded on video, and individual movements were tracked using image analysis. Next, individual movements were observed from the center of the school, in order to ensure visibility of the movements within. This point of view allowed for observations with the observer moving alongside the swarm or school to observe its interior, rather than observations from a remote location (more precisely, individual movements were observed at the center of mass of the group reference frame). When their trajectories were viewed from that perspective, the results showed that individual fish who seemed to be swimming in formation and forming a school were aggressively exchanging positions as they overlapped throughout the school. Furthermore, extended observation times showed that individuals moved entirely freely around the school, such that sometimes an individual might suddenly dart from one peripheral edge of the school to the opposite edge*11. Individuals moved around without ever stopping in fixed positions within the school, which we considered allowed for interaction with various other individuals and helped to convey information smoothly throughout the school, increasing its robustness. These results showed that unlike in mathematical models that set disturbances as originating from outside swarms, individual freedom of movement was not placed in opposition to sociality; quite the opposite, it actively contributes to swarm formation and maintenance in swarms of actual creatures.
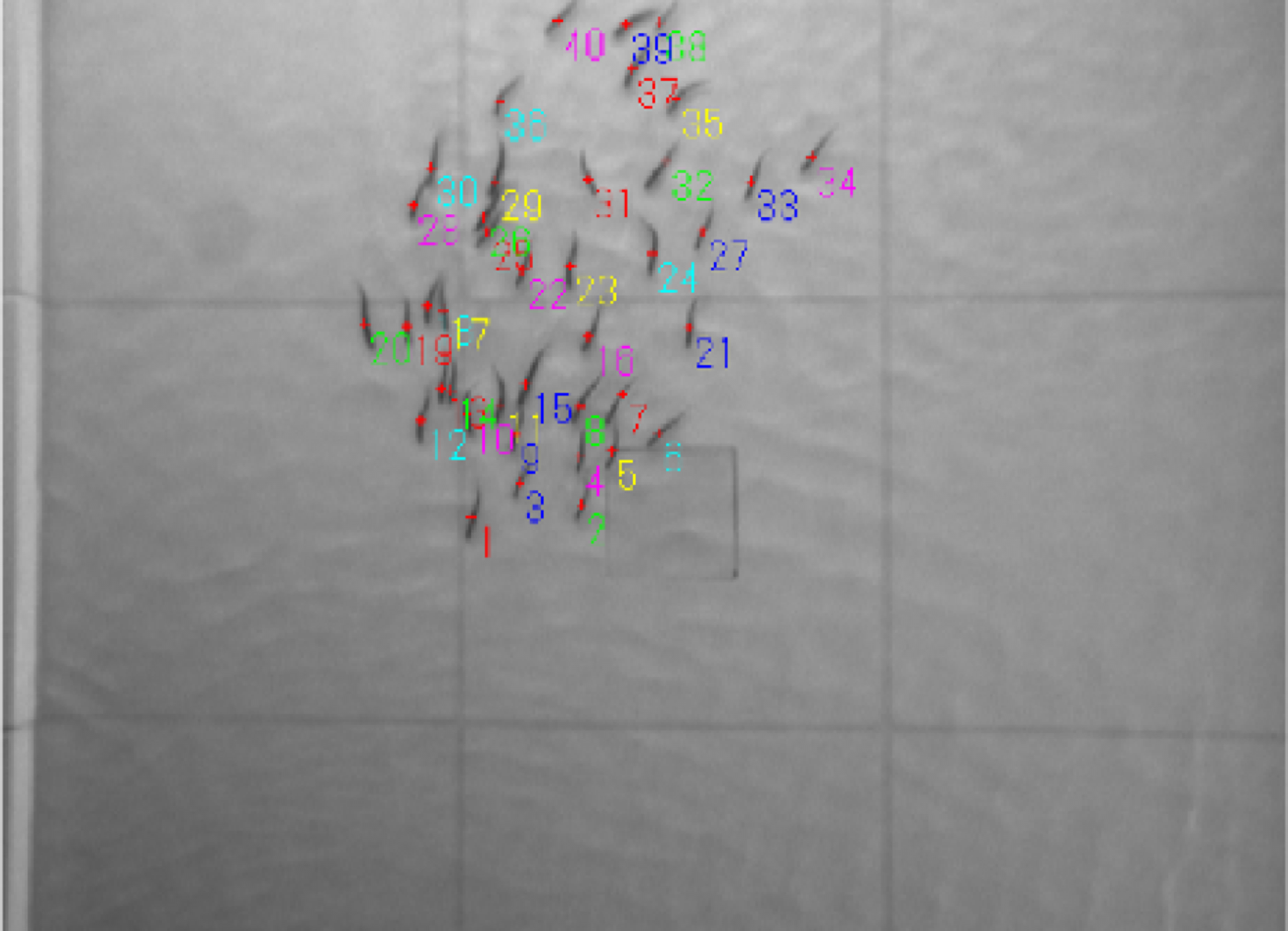
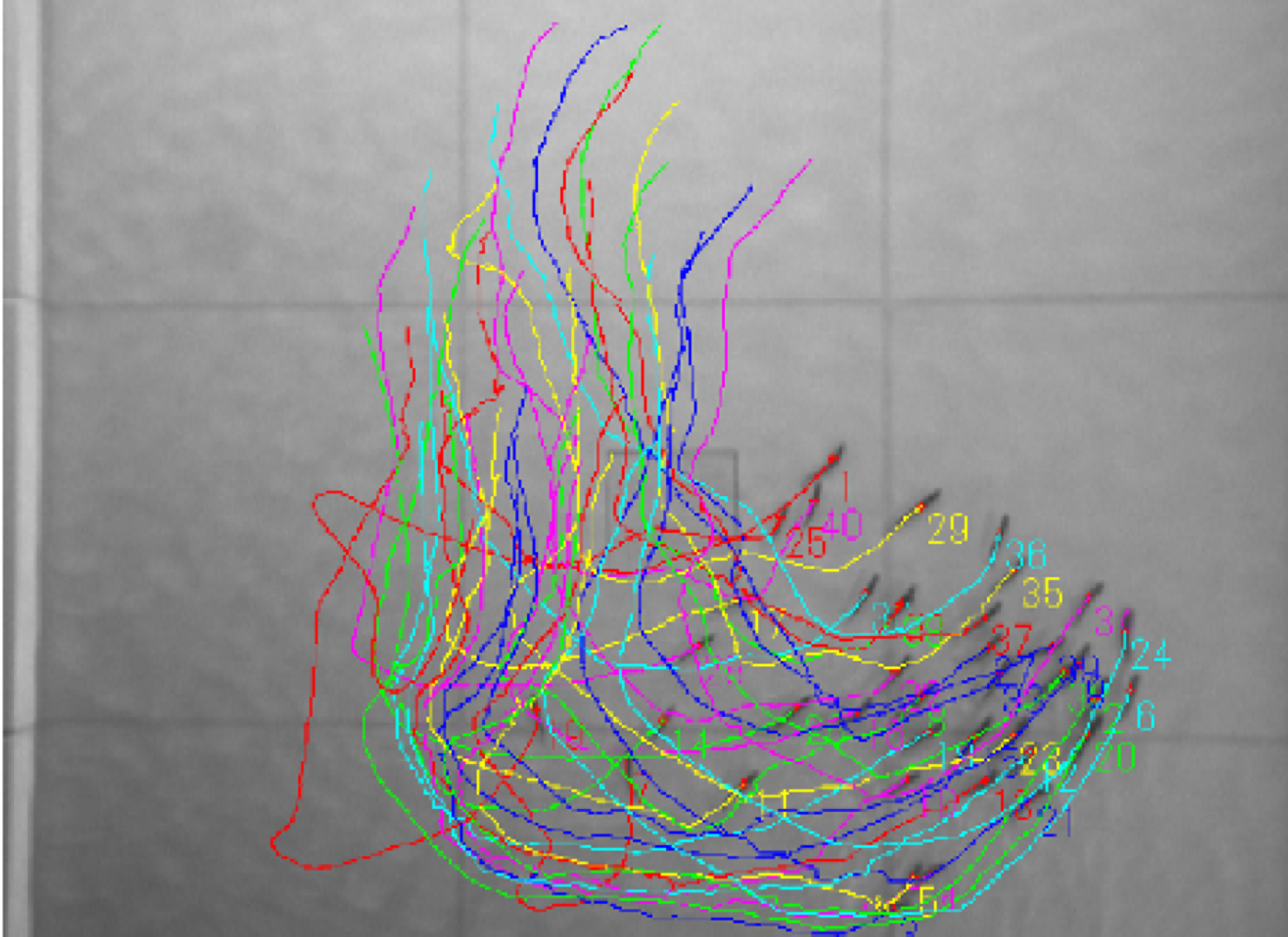
Tracking a school of juvenile ayu swimming in the experimental tank/Images by Takayuki Niizato
Asynchrony and Mutual Anticipation
Conventionally, almost all swarm models had come to assume that individuals updated their positions synchronously. But there is no way to ensure that all individuals in a swarm move in sync, just as there wouldn’t be in the aforementioned croquet example involving flamingos and hedgehogs. The behavior of objects on the constituent material level has been described with shared time as a premise, and by extension biological creature behavior has come to hold the same premise of synchronous time. But for the swarm, object behavior at the physical level is not the factor, but rather interactions and decision-making between individuals. Thus, rather than placing the regulatory mechanism for overall swarm movements outside the swarm, we should envision self-organizing swarm structures as centered on individuals pursuing asynchronous position updates within the swarm.
When examining sociability established by individuals with their own timelines, the important point to consider is interactions between individuals (including time adjustments), rather than spacial interactions like headings. The concept this gives rise to is called mutual anticipation. The individual members of swarms read and anticipate each other’s movements before acting, incorporating not only present movements, but the potential for future movement into the basis of their interactions.
Individuals engaging in mutual anticipation realize a single transition amongst multiple possible transitions, which are expressed as multiple distinct vectors. When an individual is apart from and independent of other individuals, one possible transition is selected at random from other multiple possible transitions. These independent individuals then engage in repeated irregular movements. When other individuals are nearby, the targets of their possible transitions may overlap. When multiple individuals each have overlapping the targets of possible transitions at a certain site, that site is regarded as a “popular site” or destination and each will attempt to move to it. But since the individuals move asynchronously, the first individual to move can go to that destination, while the second and subsequent individuals cannot. To avoid collisions, they move to other possible candidates. This means that an individual’s actualized possible transition interacts with other individuals’ latent (un-actualized) possible transitions through asynchronous positioning updates. Furthermore, when multiple individuals are densely swarming and possible transitions overlap among individuals throughout the swarm, each individual will have a large number of destinations. Under these conditions, in most cases, even individuals that did not get to a specific destination due to late movement can still move to another destination that remains unoccupied. Unused possible transitions from one individual’s movements contribute to the movements of other individuals to their destinations so long as these overlap with destinations that the first individual did not go to because others got there first. In other words, an individual’s un-actualized possible transition interacts with other individuals’ possible transitions as they seek to actualize it through asynchronous positioning updates.
Each individual is aggressively actively switching positions with others in the swarm. However, the swarm as a whole forms a single dense collective, and moves on its own, unilaterally. The fact that each individual has multiple possible transitions to move to creates fluctuations within the swarm. However, these inherent noises don’t threaten the swarm’s sociality; they actively help the swarm form, following principles of asynchrony and mutual anticipation. Individuals move about freely while the swarm as a whole maintains its density.
In models that forge an agreement between inherent noises and sociality, swarms are more robust against external disturbances and show scale-free correlation. These stand in contrast with other models, making them accurate reproductions of actual swarms. These models also explain the emergent phenomenon of river crossings seen in southern red crab swarms.*13。
Swarm Formation as a Structure with Consensual Breakdown
So, can we find cases where mutual anticipation and asynchrony more directly contribute to collective formation in actual swarms? There may be limits to solely observing and analyzing swarm dynamics from the interior. Addressing this, we launched a comparative study using human pedestrian swarms, intervening and altering conditions relating to mutual anticipation and asynchrony.
An experiment on the phenomenon of lane formation at a pedestrian crossing/Photo by Hisashi Murakami
Human Swarms: intervention to Mutual Anticipation
Although research into pedestrian groups originated independently from other animal collective research, it has followed a similar course*16. It began with computational model-based research. Inspired by physical particle systems, researchers arrived at a traditional collision-avoidance model, in which pedestrians repel with each other. Subsequent technological progress enabled increasing analysis of actual groups, leading to the discovery of phenomena that the traditional model could not explain. One such phenomena is anticipatory behavior. In other words, actual pedestrian behavior is impacted heavily by anticipated future positions, rather than the current positions assumed by the traditional model*17.
However, the relationship between individual anticipations and spontaneous formation of collective order remained unexamined. So, my colleagues and I conducted an experiment with 54 participants to examine crosswalk lane formation*18. Pedestrians would walk toward each other from both ends of the crosswalk. To alter the cognitive abilities of three people at the front of one group, we assigned them an additional task: they would have to solve math questions with a smartphone as they walked. This was done to distract their visual attention. It is commonly known that using a smartphone while walking narrows a pedestrian’s field of vision and significantly reduces visual attention on their surroundings. Accordingly, it was predicted that the pedestrians assigned the problem with their smartphones would be unable to pay attention to where they were going and make adequate anticipations. Analysis of individual movement trajectories showed that the collective pattern formation was significantly delayed in experiments with distracted pedestrians compared to experiments without distracted pedestrians. Further analysis at the individual level revealed that this disrupted not only pedestrians using their phones, but also those who otherwise should have had normal functioning anticipatory abilities (both those moving in the same and opposite directions of those using their phones)*19. The experimental intervention to disrupt the focus of some pedestrians had resulted not in simply distracting those specific individuals, but also indirectly impacting those around them. Conversely, it’s difficult to walk smoothly within a group when only one side is predicting the other’s behavior. The anticipation has to go mutually.
Experimental interventions affecting anticipation showed that mutual anticipation between pedestrians normally facilitates efficient pattern formation. Of course, these results are still far from fully explaining swarm phenomena. Rather, the experiment raises further questions about specific sensory inputs and other factors. Even so, these results supported mutual anticipation as a valid mechanism for swarm formation.
Human Swarms: intervention to Asynchrony
In addition to spacial-pattern formation (such as the previously examined lane formation), pedestrian groups also form temporal patterns. This appears in the synchronization of their steps. For example, in an experiment where participants walked single file in one direction in a donut-shaped experimental hallway, forming a circle*20, synchronization of participant steps could be seen at a certain density*21. Pedestrians avoided collisions by extending the same-side leg as the person ahead of them at the same time, making effective use of limited space. According to these results, synchronization of steps would seem to be a strategy for spacial resource optimization. But without the restricted conditions of an experiment, pedestrian groups walking on roads move freely with spatial self-organization. Does this spacial resource hypothesis for step synchronization hold up even under different circumstances?
To address this, we tested the spacial resource hypothesis for step synchronization in an experiment on the lane formation phenomenon*22. While the same format was used, with two groups of pedestrians on each side of a crosswalk moving toward one another, the observation method was adjusted. In conjunction with employing imaging tools to analyze trajectories, small accelerometers were fitted to both legs of the pedestrians in order to measure step synchronization. Moreover, walking was compared under both normal and altered conditions. As an added condition, an electronic metronome was played through speakers for the duration of the experiment to audibly reproduce the average walking tempo. Participants were asked to walk in sync with it. In the other scenario, participants walked normally without any conditions or audio guides.
The experimental intervention on synchronization showed that so long as no external auditory cues are given, pedestrians will not autonomously synchronize their steps. Furthermore, it showed that walking synchronization under external cues hampered the variety of pedestrians’ lateral movement, made movements simpler, and led to the formation of fragile spacial structures. Externally synchronized groups often formed narrow lines. These conditions increased the amount of contact with the lane moving the other direction, and led to major structural disruptions from even slight external disturbances. In contrast, regular pedestrian groups without audio guides were more robust, but did not align their steps. The premise on which spacial resource conservation theories of step synchronization were built was concerned with achieving synchronization, but this experimental intervention suggests that it would be better to focus on asynchronous movement to evaluate its contributions to the robustness of group formation. For example, even in phenomena that might seem like coordinated behavior, the disjunction between the timing at which two people move to go to the same location may be the result of one person adjusting to give the other person time to go in another direction. Although more detailed analysis is required, in any case, it seems likely that the individual movements that presage collective movement are asynchronous to begin with, and that considering the contributions to group robustness from freedom and diversity of individual movement and its asynchrony strikes closer to the heart of swarm issues.
Swarms inherently generate noises
As this paper has outlined, a swarm without a state to return to cannot be described as a stable system. Rather, swarms are considered a robust system in which the internal disturbances that occur actively reinforce the formation of the swarm. In robust systems, internal and external disturbances cannot be distinguished. Precisely because they are open systems where internal and external are indistinguishable, swarms form systems that are extremely difficult to break apart even as they undergo constant change. That is why it is critical to observe swarms as open systems undergoing constant generation, given their inundation with disturbances that fundamentally defy definition as closed systems.
Goro Murayama, Decoy-walking, 2019
“You’d be amazed how much it takes for me to be myself”
I don’t go to a lot of academic conferences and don’t have all that many acquaintances, but looking back, I feel as though I always go around picking up the tail ends of conversations. I am not sure if these experiences will stimulate me in one way or another and provide some sort of insight. Even so, I sometimes feel as though these cut-off conversational fragments end up connecting with other fragments, over time.
At that particular conference, I was presenting the results of my summer 2023 study on Iriomote Island, which showed that fiddler crabs*26 have two kinds of memories for locating their burrows. The two memories I presented from the sum of the data are perhaps best broken down into object-level memory (memory of the burrow location as such) and meta-level memory (for evaluating the certainty of that memory). When investigating animal meta-cognition, researchers often make the animals memorize symbols with minimal relationships to animal ecosystems, then make them select the memorized symbols from amongst other symbols (distractors). The animals get treats for answering correctly, and nothing for the wrong answer. There are also other symbols for avoiding making a decision. When an animal chooses to avoid a decision, it gets a worse treat than for a correct answer, but still a treat. This sort of experimental design investigates whether animals can avoid making a choice appropriately when they are uncertain about their memories. The ability to pass this test indicates whether an animal is capable of “human-like” metacognition. However, the design of this test for meta-cognition requires the separation of animals that have it (to a certain point) and those who do not. In short, it leads to a finding that meta-cognition began with one species of animals alone, suddenly, over the course of evolution. But does that lead to an inquiry into the origins of meta-cognition? Wouldn’t one need to perform an experiment that touched on the ecological context (including the animal’s body) in order to directly address the system at which meta-cognition occurs? That was the theme of my presentation.
Bateson argues that learning progresses poorly in the absence of context that provides a framework for it*27, because the significance of things reinforced through learning can vary with context. The same could be said of memory, too. There is no way to use memory in the first place without the context to frame it and the meta-cognition to evaluate its reliability, and the uses for memories can vary depending on the memory’s certainty. Evaluating the certainty of memory contexts can change according to social interactions. For example, assume that there’s a memory that becomes unreliable due to social interactions with others that are not directly related to that memory. When that happens, the context for estimating the certainty of the original memory is tied up with interactions with others, which makes the memory uncertain. (note that estimated memories do not always align with actual memory accuracy. There can be “illusions” that cause disagreements between the two.)
As Bateson already argues, context is accompanied by more and more context (such as the fundamental social relations with others on which social interactions are premised), and that by still more context (such as the routinely-shifting spacial relationships of routine environments, upon which social relations are premised), extending endlessly into the unknown. There is a deeply rooted tendency to assume that the meta-cognition that identifies context is an independent, self-referential system. But it isn’t possible to remove actual meta-cognition from the context in which it functions. When re-envisioned this way, meta-cognition that incorporates even the ecosystem can be re-defined as a system with latent but deep-seated dynamism ultimately arising out of externally-applicable context that cannot be pre-assumed.
It would be the same of self-consciousness, in which having a characteristic open to change is inextricably linked to an endlessly expansive context, which encompasses all externalities down to the ecosystem. In the end of Ghost in the Shell, the Major merges with the Puppet Master. At that point it seems to me as though the Major’s existential anxiety about the outline of the self vanishes, as the being’s transcendent form, derived from the Puppet Master, allows it to perceive the entire ocean of the net. It seems clear that the Puppet Master’s answer to the Major’s question of ” What guarantee is there that l’ll remain ‘’me’’?” is that “None” and the merger is her reaction to this. Likewise, the Puppet Master is correct in their response that “But to be human is to continually change”. It seems that “change” is assured even after this fusion. However, isn’t the “change” in this case just a form of growth, in which an established, defined entity with an explicit outline expands by taking on what it desires? Even though it changes, its baseline state remains assured and its existence remains stable. At the same time, other changes are also conceivable. This is to say that because the system has no set form, inherently generates disturbances, and encompasses endless disjunctions, it can experience robust, unintended changes not expected from external relationships. I can’t help but feel that such changes have been overlooked. And that is precisely why I always feel a sense of lingering loneliness when I see the Major/Puppet Master setting out on a journey into the vast net, devoid of any shade of anxiety or unease in their final post-merger scene.
[Notes]*1
Take for example a rubber prosthetic left hand that is placed on a table, with the participant’s actual left hand placed to the left of that. There is a divider between them, hiding the participant’s hand but leaving the rubber hand visible. Leaving the hands in this state, both the rubber and real hand are given tactile stimulation at the same time (participants are instructed to look at the rubber hand as this happens). This will cause the participant to begin to feel as though the rubber hand is theirs (ownership transfers to the rubber hand). This sensory illusion is believed to occur due to synchronization between vision and touch.
Botvinick, M. and Cohen, J. (1998). Rubber hands ‘feel’ touch that eyes see. Nature, 391, 756. Experimental results contradicting this explanation have also been reported.
Rohde, M., Di Luca, M., & Ernst, M. O. (2011). The Rubber Hand Illusion: Feeling of Ownership and Proprioceptive Drift Do Not Go Hand in Hand. PloS one, 6(6), e21659.Out-of-body experience sensory illusions are one full-body expansion of this rubber hand illusion.
Lenggenhager, B., Tadi, T., Metzinger, T., & Blanke, O. (2007). Video Ergo Sum: Manipulating Bodily Self-Consciousness. Science, 317(5841), 1096-1099.*2
Nishiyama, Y., Yamashita, C., & Nomura, S. (2023). An illusion of disownership over one’s own limb is associated with pain perception. Scientific Reports, 13(1), 2801. *3
Couzin, I.D. (2007). Collective minds. Nature, 445, 715. *4
Reynolds, C. W. (1987, August). Flocks, herds and schools: A distributed behavioral model. In Proceedings of the 14th annual conference on Computer graphics and interactive techniques (pp. 25-34). *5
Vicsek, T., Czirók, A., Ben-Jacob, E., Cohen, I., & Shochet, O. (1995). Novel type of phase transition in a system of self-driven particles. Physical review letters, 75(6), 1226. Details on other swarm models are as follows.
Murakami, H., Abe, M. S., & Nishiyama, Y. (2023). Toward Comparative Collective Behavior to Discover Fundamental Mechanisms Underlying Behavior in Human Crowds and Nonhuman Animal Groups. Journal of Robotics and Mechatronics, 35(4), 922-930. *6
Cavagna, A., Cimarelli, A., Giardina, I., Parisi, G., Santagati, R., Stefanini, F., & Viale, M. (2010). Scale-free correlations in starling flocks. Proceedings of the National Academy of Sciences, 107(26), 11865-11870. *7
An endemic Japanese species native to river estuaries in the Ryukyu archipelago. A rarity among crabs, they walk forward instead of sideways (the latest research indicates that originally forward-walking ancestors evolved for a time into sideway-walking crabs before reverting back into forward-walkers; Takuya Taniguchi, Tsubasa Inoue, Jung-Fu Huang, Atsushi Hirai, Nobuaki Mizumoto, Fumio Takeshita, Yuuki Kawabata. “How did locomotion in crabs evolve?: Restoration of ancestral forms using current speciation data”. 42nd Annual Conference of the Japan Ethological Society). The crabs are called “soldier crabs” also in Japanese due to the massive swarms they form to silently advance across the shore. Given that an adult’s shell is slightly larger than a bead and turn a brilliant blue in winter – despite darkening to black under the intense summer sunlight (Jinno, M., Doi, W., Mizutani, A., & Kohno, H. (2020). Seasonal changes in body color of Mictyris guinotae (Brachyura: Mictyridae). Crustacean Research, 49, 133-140), they are also sometimes called blue jewels. They dig into the sand to hide when threatened, then begin emerging and swarming on the surface after some time passes.*8
Murakami, H., Tomaru, T., Nishiyama, Y., Moriyama, T., Niizato, T., & Gunji, Y. P. (2014). Emergent runaway into an avoidance area in a swarm of soldier crabs. PloS one, 9(5), e97870; Murakami, H., Tomaru, T., Niizato, T., Nishiyama, Y., Sonoda, K., Moriyama, T., & Gunji, Y. P. (2015). Collective behavior of soldier crab swarm in both ring-and round-shaped arenas. Artificial Life and Robotics, 20, 315-319. *9
In Japan, sweetfish are known to require a specific style of fishing to catch. As the style teaches, sweetfish exhibit territorial and mutually exclusive behavior as adults, while as juveniles they form large schools and exhibit textbook swarm behavior in the same manner as schools of sardines.*10
This research is compiled into the following paper co-authored with Assistant Professor Takayuki Niizato of the University of Tsukuba.
Murakami, H., Niizato, T., Tomaru, T., Nishiyama, Y., & Gunji, Y. P. (2015). Inherent noise appears as a Lévy walk in fish schools. Scientific reports, 5(1), 10605. *11
This sort of internal individual behavior on the inside of a swarm has been found to follow a movement pattern known as Lévy walk, which have no specific scale (with distribution according to power laws) and which connect clusters of many short steps with rare long steps. From this movement, it is believed that each individual takes advantage (exploiting) interactions with other individuals nearby, while also exploring interactions with new individuals at all times. Furthermore, Lévy walk movement is a strategy seen in various animal searching (foraging, in particular) behavior, and is known for optimizing searching efficiency for resources distributed in unpredictable ways. In recent years, its functional advantages have become clear, in the form of flexibility of actions beyond exploratory strategies (Abe, M.S. (2020) Functional advantages of Lévy walks emerging near a critical point, Proceedings of the National Academy of Sciences of the United States of America, 117(39), 24336-24344).*12
Research conducted under my PhD supervisor, Professor Yukio-Pegio Gunji (currently at Waseda University).
Gunji, Y. P., Murakami, H., Niizato, T., Adamatzky, A., Nishiyama, Y., Enomoto, K., Toda, M., Moriyama, T., Matsui, T., Iizuka, K. (2011) Embodied swarming based on back propagation through time shows water-crossing, hourglass and logic-gate behaviors. In ECAL 2011: The 11th European Conference on Artificial Life. MIT press; Murakami, H., Niizato, T., & Gunji, Y. P. (2012). A model of scale-free proportion based on mutual anticipation. International Journal of Artificial Life Research (IJALR), 3(1), 34-44; Murakami, H., Niizato, T., & Gunji, Y. P. (2017). Emergence of a coherent and cohesive swarm based on mutual anticipation. Scientific reports, 7(1), 46447. *13
Considering that anticipation is believed to take place collectively, taking a model based on asynchrony and mutual anticipation allows us to describe behavior possible only by a swarm (and not by a single individual). One example would be soldier crabs crossing rivers. At low tide, the crabs come out of the mud and move around the tidal flats, but never try to enter the rivers and pools alone. However, when they form large enough groups with a high enough density, they suddenly will start entering the water and crossing rivers and pools. This emergent phenomenon is difficult to explain with standard models, but is easy to reproduce with interaction models. As described previously, when the targets of possible transitions of multiple individuals overlap at a site, it becomes the swarm’s destination. But in this case water areas are taken as special, and defined as requiring more overlap for a destination before it becomes the swarm’s destination. This in turn shows that groups with fewer individuals will not enter the water due to lacking adequate overlapping, but larger groups can form dense collectives in water due to there being more transitions capable of overlapping. This lets them enter the water as a swarm.*14
In particular, Niizato, T., Murakami, H., & Musha, T. (2023). Functional duality in group criticality via ambiguous interactions. PLOS Computational Biology, 19(2), e1010869. *15
Murakami, H., Feliciani, C., & Nishinari, K. (2019). Lévy walk process in self-organization of pedestrian crowds. Journal of The Royal Society Interface, 16(153), 20180939 shows other behavior similar to Lévy walks in pedestrians forming lanes.*16
Historical research on pedestrian crowd behavior is compiled into the aforementioned Murakami, H., Abe, M. S., & Nishiyama, Y. (2023).*17
Traditional models considered there to be an effect like the two poles of a magnet that would repel two pedestrians from one another more strongly the closer they were to each other, and which faded away with distance. Thus, traditional models emphasized the relationship between positions at the current time. However, analysis of pedestrian behavior in a wide range of walkway and sidewalk scenarios showed that pedestrian behavior is impacted heavily by anticipated positions rather than current positions. Naturally, it is easy to foresee an eventual collision between two pedestrians on a busy street even if they are walking at a distance, so long as they are each walking toward the other. In such instances, both parties will try to avoid this before it happens. Alternately, even if we assume they’re fairly close together, there is no risk of two pedestrians colliding so long as they are both walking in the same direction. No one takes action to avoid anyone else in that scenario.*18
Murakami, H., Feliciani, C., Nishiyama, Y., & Nishinari, K. (2021). Mutual anticipation can contribute to self-organization in human crowds. Science Advances, 7(12), eabe7758. *19
Pedestrians paying attention to their destinations and anticipating events will show collision avoidance behaviors even if an oncoming pedestrian is at an adequate distance. However, pedestrians under restrictions with diverted focus are difficult to anticipate, and we can predict that avoidance will take the form of a sudden, sharp turn at close range. When the actual frequency of these sudden sharp turns was examined, it was found to be more common under conditions of interrupted anticipation. However, it bears mentioning that avoidance via sharp turns occurred in both the phone users and other pedestrians alike.*20
Similar experimental configurations are commonly used to investigate relationships between factors such as minimum formats (such as interactions between two people walking one ahead of the other) and densities for inter-pedestrian interactions. This configuration is used in other experiments to investigate the phenomenon of traffic jams, and has an advantage of being easily explained with models such as the cellular automaton model. For an example, see Nishinari, Katsuhiro. Traffic Jam Studies. (Shinchosha, 2006).*21
Ma, Y., Lee, E. W. M., Shi, M., & Yuen, R. K. K. (2021). Spontaneous synchronization of motion in pedestrian crowds of different densities. Nature human behaviour, 5(4), 447-457. *22
This research has been summarized in my jointly-corresponding-authored papers with Dr. Takeyoshi Tomaru.
Tomaru, T., Nishiyama, Y., Feliciani, C., & Murakami, H. (2023). Robust spatial self-organization in crowds of asynchronous pedestrians. bioRxiv, 2023-08. (preprint)*23
Shitone Sakamaki (2023), “VerbFeS manifesto“*24
Jen E. (2003) Stable or robust? What’s the difference? Complexity, 8(3), 12–18.*25
Gunji, Y. P., Murakami, H., Niizato, T., Nishiyama, Y., Tomaru, T., & Adamatzky, A. (2012). Robust swarm model based on mutual anticipation: swarm as a mobile network analyzed by rough set lattice. International Journal of Artificial Life Research (IJALR), 3(1), 45-58. *26
My colleagues and I studied Okinawan Austruca perplexa fiddler crabs. Only males of the fiddler crab family have the major claw much that is larger than the minor claw and is the same size as the rest of their shells, showing extreme sexual dimorphism. The species is known to use the gigantic claw in a variety of social behaviors (such as mating displays and territorial disputes). The Japanese name for fiddler crabs (Shiomaneki) references calling in the tides, and comes from the way their mating displays of waving their claws looked as though they are beckoning the tide. Their English name is similar, and comes from the motion of playing the violin or fiddle. Specifically, it references the way these crabs feed by using their smaller claw to frequently bring mud to their mouths, resembling someone moving a violin bow (in this case their larger claw). (https://www.fiddlercrab.info) While the English name notes precise details of the species, it ignores the issue of their relationship with the environment, and could be taken as simply anthropomorphizing them with human-like behavior. Between the two, I would have to express my preference for the Japanese name. The species is also known for its incredibly refined spacial processing abilities.
Murakami, H., Tomaru, T., & Gunji, Y. P. (2018). Exclusive shift from path integration to visual cues during the rapid escape run of fiddler crabs. Animal Behaviour, 144, 147-152; Murakami, H., Tomaru, T., & Gunji, Y. P. (2017). Interaction between path integration and visual orientation during the homing run of fiddler crabs. Royal Society open science, 4(9), 170954. *27
Gregory Bateson, “Steps to an Ecology of Mind” vol. 2 (trans. Yoshiaki Sato), Iwanami Bunko, 2023. pp. 174, 205, 274-275.
Hisashi Murakami
Born in Osaka prefecture in 1987. Murakami became involved in collective animal behavior research after joining in professor Yukio Gunji’s lab at the Kobe University Faculty of Science. After completing his PhD at the same university, he worked as a researcher at Waseda University, as an assistant professor at Kanagawa University, and as a project assistant professor at the University of Tokyo before taking a position as an assistant professor at the Kyoto Institute of Technology in January 2021. He received the Ig Nobel Prize in kinetics in September 2021 for research conducted jointly with Nagaoka University of Technology associate professor Yuta Nishiyama, University of Tokyo project associate professor Claudio Feliciani, and professor Katsuhiro Nishinari.